Neurovascular surgery: a review of the way forward from the American perspective
Introduction
Neurovascular surgery is a complex surgical subspecialty that involves diagnosing and treating vascular lesions of the brain and spinal cord using endovascular and microsurgical techniques. In the United States (U.S.), neurovascular disorders are a public health crisis and impose substantial healthcare costs, impacting the lives of millions (1,2). However, neurovascular surgery in the U.S. has transformed dramatically over the past few decades, driven by rapid advancements in endovascular approaches and imaging modalities towards precise and effective treatment of a variety of conditions such as stroke, aneurysms, arteriovenous malformations (AVMs), and chronic subdural hematomas (cSDHs) (3). Today, the U.S. neuroendovascular workforce comprises neurosurgeons, neurologists, and radiologists who have undergone extensive training to serve the increasing burden of cerebrovascular disease (4,5), while open neurovascular surgery remains the fiefdom of vascular neurosurgeons. With endovascular procedures now constituting approximately 75% of vascular neurosurgeons’ practice (6), the field is marked by a shift toward minimally invasive neuroendovascular methods that are both safe and highly effective, aligning with patient preferences for less invasive interventions, reserving open surgery for complex cases where endovascular methods are unsuitable, or if complications arise from endovascular procedures (7). Despite these advancements, the field of neurovascular surgery faces challenges, including regional disparities in access to neurovascular interventions and the declining case volumes for open surgical techniques, which impacts training and skill transfer between open and endovascular techniques.
While numerous reviews have explored the advancements in neurovascular surgery, most focus on specific innovations or global trends without emphasizing the unique challenges and opportunities within the U.S. healthcare system. This review seeks to bridge that gap by providing a comprehensive overview of the evolution of neurovascular surgery in the U.S., with a focus on training pathways, workforce trends, and key technological advances. Differentiating itself from prior literature, this review also addresses the challenges posed by regional disparities in access to care and declining open surgical case volumes, offering strategies for sustaining a balanced neurovascular workforce. Furthermore, it provides a forward-looking perspective on emerging technologies, such as artificial intelligence (AI), robotic systems, and augmented reality (AR), and how they can address current limitations.
Methods: literature search
Key databases, including PubMed, Embase, and Web of Science, were searched for articles published from January 2000 to December 2024. Search terms included “neurovascular surgery OR neuroendovascular surgery”, “endovascular techniques”, “microsurgery OR open neurovascular surgery”, “training in vascular neurosurgery”, “artificial intelligence in surgery”, “robotic-assisted neurovascular surgery”, and “advances in neurovascular surgery”. Inclusion criteria focused on studies relevant to the U.S. healthcare context, though selected articles from high-income countries were included for comparison. References from identified studies were also reviewed to ensure completeness.
Current state of neurovascular surgery in the U.S.
Advances in endovascular techniques
The development of endovascular techniques has caused a shift towards minimally invasive neurointerventions for cerebrovascular disease, leading to improved patient care. The merits of neuroendovascular interventions are evidenced by their use in a myriad of neurovascular conditions, including intracranial aneurysms, AVMs, dural arteriovenous fistulas (dAVFs), cavernous malformations, cSDHs, stroke, and tumors. This is paralleled by advances in endovascular devices like intracranial stents, detachable coils, detachable balloons, flexible catheters, steerable wires, intravascular (flow diverter) stents, and intrasaccular devices.
Intracranial aneurysms
Endovascular treatment of intracranial aneurysms historically involved the use of coiling and stenting methods, with advances primarily aimed at improving the structure and packing density of these devices (8). However, these methods excluded management of challenging intracranial aneurysms like wide neck and bifurcation aneurysms. The introduction of stent-assisted coiling, including the use of single stents and Y-stenting techniques, provided a significant step forward in addressing these challenging cases. Intrasaccular devices, such as the Woven EndoBridge (WEB), further revolutionized treatment by disrupting intra-aneurysmal flow while minimizing metal coverage of the parent vessel (9). The Contour device is a mixed flow diversion-flow disruption device also used in the treatment of wide-neck aneurysms. With reported 1-year occlusion rates of 54% and 56% in the WEB and Contour devices, respectively (10,11), the development of more effective devices is necessary. Most recently, the Saccular Endovascular Aneurysm Embolization System (SEAL) and Trenza devices have emerged, further enhancing the efficacy and safety of endovascular interventions for complex aneurysms. The SEAL strategy has achieved 3-month partial and complete obliteration rates of 81.5% and 74.1%, respectively, while Trenza has achieved a preliminary 6-month adequate occlusion rate of 83% (12). Flow diversion has largely replaced previous endovascular and surgical treatments for intracranial aneurysms following results of the Pipeline for Uncoilable or Failed Aneurysms Study (PUFS) trial, which reported complete aneurysm obliteration rates at 90% within the first year (13). Utilizing a mechanism that treats the parent vessel extrinsic of the aneurysm dome, flow diverters are routinely used in the management of giant and complex aneurysms with increased safety and efficacy (14,15). Flow diverters are limited by their requirement of dual-antiplatelet therapy, with advances focused on surface modifications to mitigate this thrombogenicity risk (16). The use of flow diverters in challenging vascular territories like the vertebrobasilar system, although efficacious, carries the risk of occluding perforating vessels, necessitating caution (17,18).
Early results from the PRE-SEAL IT trial that have been presented at multiple conferences are encouraging, although there has been no formal publication to date. For the 33 total treated aneurysms, the 3-month follow-up showed 74.1% complete occlusion and 81.5% adequate occlusion (12).
Stroke
Developments in endovascular techniques have shifted the paradigm of stroke treatment from a medical disease to a surgical one. Starting from the first mechanical thrombectomy device, MERCI, approved by the Food and Drug Administration (FDA) in 2004, significant neuroendovascular innovations led to second generation stent retriever and aspiration catheter devices (19). These devices led to improved stroke care thorough improved revascularization rates, expanded stroke treatment windows, and liberal patient eligibility for intervention. The clear benefits of endovascular thrombectomy trials led to early termination of these trials as clinical equipoise was met, with the American Heart Association (AHA)/American Stroke Association (ASA) guidelines recommending endovascular thrombectomy as a putative treatment strategy for ischemic stroke caused by large vessel occlusion (LVO) (20). Accordingly, advances in endovascular thrombectomy devices have accompanied this revolution in stroke care (21-23). The importance of mechanical thrombectomy in the U.S. is exemplified by the shift in stroke center certification following its introduction, using guidelines and recommendations by the AHA/ASA, Brain Attack Coalition (BAC), and The Joint Commission (TJC) (24). Currently, three accrediting bodies in the U.S., TJC, the Det Norske Veritas (DNV), and the Accreditation Commission for Health Care (ACHC), describe four tiers of stroke centers: (I) acute stroke ready hospitals (ASRHs), which can properly identify stroke patients and provide them with initial treatment prior to transfer to a more advanced center; (II) primary stroke centers (PSCs), which can provide medical management (e.g., intravenous thrombolysis) to appropriately evaluated stroke patients; (III) thrombectomy-capable stroke centers (TSCs), also known as PSC plus centers, are those that can provide mechanical thrombectomy to stroke patients; and (IV) comprehensive stroke centers (CSCs), which in addition to possessing advanced neuroimaging facilities and endovascular neurointerventional capabilities, engage in patient-centered and epidemiological stroke research, and open surgery (25). In 2018, 2,446 stroke centers were recorded in the U.S., of which 678 were ASRHs, 1,459 PSCs, 12 TSCs, and 297 CSCs (24). It is also estimated that the number of thrombectomy procedures for acute ischemic strokes will grow at a rate of 5–10% annually (5). Currently, 526 U.S. stroke centers provide neurovascular services, defined as TSCs and CSCs, across 48 states, with the highest counts seen in California (n=65), Florida (n=61), Utah (n=52) and New York (n=31) (Figure 1, Table 1, and the online table available at https://cdn.amegroups.cn/static/public/asj-24-45-1.pdf). Approximately 24% to 46% of ischemic strokes in the U.S. are attributed to LVO (26), yet only 27% of these patients have access to thrombectomy due to limitations in the distribution of stroke centers, workflow inefficiencies, and the availability of a trained neurovascular workforce. Consequently, two-thirds of eligible patients are unable to receive this life-saving intervention (27,28).
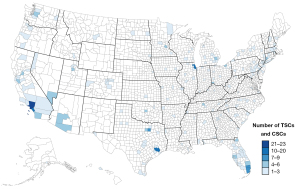
Table 1
Variables | CSC (n=397) | TSC (n=129) | Total (n=526) |
---|---|---|---|
Region | |||
Northeast | 59 | 28 | 87 |
Midwest | 89 | 25 | 114 |
South | 170 | 36 | 206 |
West | 79 | 40 | 119 |
Accreditation body | |||
ACHC | 10 | 5 | 15 |
DVN | 175 | 28 | 203 |
TJC | 212 | 96 | 308 |
ACHC, Accreditation Commission for Health Care; CSC, comprehensive stroke center; DNV, Det Norske Veritas; TJC, The Joint Commission; TSC, thrombectomy-capable stroke center.
Hydrocephalus
A recent discovery in the anatomic relationship between the cerebellopontine cistern and inferior petrosal sinus has led to an endovascular approach to treating hydrocephalus, through creation of a cerebrospinal fluid (CSF)-venous fistula for CSF diversion (29). The first of such an approach, using the eShunt device transvenously, have had their first reports in the U.S. for communicating hydrocephalus by mimicking an arachnoid granulation for CSF shunting (29,30). This endovascular approach presents a minimally invasive approach with less infection and device failure risks, compared to current techniques of ventricular shunting and endoscopic third ventriculostomy. Deployment of the eShunt device requires careful planning, high-resolution imaging, and endovascular dexterity given the extensive vascular environment of the inferior petrosal sinus (31). The eShunt is a new direction in hydrocephalus treatment that requires more clinical data and will undoubtedly undergo device innovation as with other neuroendovascular systems.
cSDH
The aging and comorbid population has led to an increased incidence of cSDH (32), which initially treated by surgical craniotomy and drainage, has evolved towards endovascular devascularization. Endovascular treatment of cSDH involves the embolization of the middle meningeal artery (MMA), through particle and liquid embolic agents, to mitigate the abnormal MMA vascularity of the subdural membranes and capillaries, as well as prevent disease progression (33). While existing surgical strategies recur in up to 25% of patients, MMA embolization as standalone treatment or as adjunct to surgery, results in lower recurrence rates (34,35).
AVMs
Brain AVMs have long been treated with neuroendovascular techniques, though advances have focused on better embolic agents and devices, treatment approaches, and patient selection. Device innovations in AVM treatment have included embolic agents for improved AVM nidal penetration and improved catheter and microwire designs for distal catheterization (36-38). AVM treatment in the U.S. is currently multimodal, including observation, and combining neoadjuvant embolization with open surgery, or (neo)adjuvant embolization with radiosurgery (39). The transvenous approach to AVM embolization, although initially associated with high rates of hemorrhagic complications, is slowly increasing in popularity as standalone AVM treatment among neuroendovascular surgeons as familiarity with the technique increases with a shift towards improved safety of this technique (40)
Carotid-cavernous fistulas (CCFs) and dAVFs
CCFs have evolved to be mostly treated by endovascular or radiosurgical strategies. Endovascular embolization approaches can be transvenous or transarterial (41). Transarterial embolization, via small meningeal branches supplying the fistula, is used for direct, high-flow CCFs (42). Following the removal of detachable balloons from the U.S. market in 2003 (43), advances in transarterial embolization have evolved to include platinum coils, embolic materials, and covered stents, which have shown to be effective in CCF obliteration (41). The transvenous embolization approach is commonly used for CCFs inaccessible via the transarterial approach. This route commonly uses the inferior petrosal sinus and superior orbital vein to reach the cavernous sinus (41), with a reported high CCF obliteration rate with coils and embolic agents. A recent meta-analysis demonstrated comparable efficacy between the transarterial and transvenous approaches to CCF embolization, with appropriate patient and CCF selection criteria (44). Flow diverters have been used to treat direct CCFs and dAVFs often in conjunction with other embolic agents (45). However, more studies are needed to incorporate this into clinical practice.
Like CCFs, dAVFs are also treated with transarterial and transvenous endovascular approaches. The transarterial approach is mainly used for high-grade dAVFs with the use of liquid embolization agents, which can be injected through dual-lumen balloon catheters to facilitate deep fistula penetration (37,46). Transvenous approaches to dAVFs are used in cases where arterial access is difficult and unsafe, such as in cases where the dAVF is supplied by small tortuous arteries or where dangerous anastomoses are present (47). This approach may utilize coils or liquid embolic agents, using the femoral and internal jugular veins, or by direct puncture (46).
Idiopathic intracranial hypertension (IIH)
Venous sinus stenting is an endovascular treatment increasingly gaining recognition as a treatment strategy for IIH in cases where cerebral venous sinus stenosis is the underlying etiology (48). Indications for venous sinus stenting include both the presence of radiographic findings of venous sinus stenosis and physiologic evidence of a venous sinus pressure gradient (49). Given the risks of complications of the procedure and the requirement for post-operative antiplatelet/anticoagulant therapy, appropriate patient selection is paramount (50). Venous sinus stenting also shows clinical benefits in patients with pulsatile tinnitus caused by venous stenosis (51).
Advances in microsurgical techniques
The practice of open neurovascular surgery has not been met with the degree of advancements seen in neuroendovascular techniques, leading to a reduction in microsurgical case volume. While innovations in the neuroendovascular space have moved towards faster, easier, and safer approaches, open neurovascular techniques remain technically difficult, requiring meticulous dexterity, and are reserved for complex lesions not amenable to neuroendovascular approaches (51,52). The current landscape of open neurovascular surgery in the U.S. includes treatment of intracranial aneurysms, vascular malformations, carotid atherosclerotic disease, and intracerebral hemorrhage.
Intracranial aneurysms
Most intracranial aneurysms are now treated by endovascular techniques, leaving complex aneurysms to microsurgery. Aneurysms that fit this bill include wide-necked aneurysms, large saccular aneurysms with multiple efferent arteries, dolichoectatic aneurysms, previously endovascularly treated aneurysms, and aneurysms with atherosclerotic lumens (53,54). These aneurysms are treated using bypass, clipping, vessel reconstruction, and trapping techniques. Extracranial-intracranial (EC-IC) bypasses with aneurysm trapping can use either scalp arteries like the superficial temporal artery (STA) connected to the middle cerebral artery (STA-MCA bypass) or an interpositional graft (e.g., the saphenous vein or radial artery) between the distal external carotid artery and the supraclinoid internal carotid artery or MCA to double bypass flow (53,55). Alternatively, intracranial-intracranial (IC-IC) bypasses, involving excision of the diseased vascular segment with direct vessel reconstruction, are challenging techniques useful for treating complex anterior cerebral artery (ACA) and posterior inferior cerebellar artery (PICA) aneurysms (55). These bypasses are shorter in length, do not require extracranial donor arteries, and are protected from external trauma (56). Open surgery may also be used as adjunct to endovascular techniques, such as combined clipping and coiling in ruptured blister aneurysms, characterized by ill-defined necks and fragile walls (57), or microsurgical rescue in cases where endovascular treatment fails (58).
Vascular malformations
Microsurgery remains the mainstay treatment for brain AVMs in the U.S., with the highest cure rates and lowest complication rates compared to other treatment modalities (39,59). However, it may be limited by prolonged hospitalization and longer recovery times. Given the heterogenous nature of these lesions, appropriate patient selection is pertinent in the microsurgical treatment of brain AVMs, with recommendations centered on standalone microsurgery for low-grade lesions (Speltzer-Martin grades I and II), conservative treatment for high-grade lesions (Speltzer-Martin grades IV and V), and more research required for defining patient selection for intermediate-grade lesions (Speltzer-Martin grade III) that focuses on lesion-specific characteristics (60,61). Whereas AVM embolization is mainstay in Europe for ruptured AVMs, followed by radiosurgery, and finally microsurgical resection for refractory lesions (36), the findings from the TOBAS study suggest reservation of embolization only in cases where microsurgery is likely to be beneficial (62).
Surgical treatment for cerebral cavernous malformations (CCMs) is rare and reserved for cases where the CCM cannot be accessed through endovascular techniques (63). The location of CCMs is also important, with seemingly inoperable brainstem lesions having undergone microsurgical advances to recommendation of open surgical resection as first-line treatment for some brainstem CCMs (54).
The American pathway to becoming a neurovascular surgeon
Training in the U.S. in neurovascular surgery can be undertaken by physicians completing their residency in neurosurgery, neurology, and radiology for neuroendovascular fellowships, and neurosurgery for open vascular fellowships. Guidelines for the training curriculums are provided by multidisciplinary teams that include the Accreditation Council for Graduate Medical Education (ACGME), the Joint Section of Cerebrovascular Surgery (JSCVS) for the American Association of Neurological Surgery (AANS) and Congress of Neurological Surgeons (CNS), the Society of Neurointerventional Surgery (SNIS), and the Society of Vascular and Interventional Neurology (SVIN). Additionally, the Committee on Advanced Subspecialty Training (CAST) has also been created to oversee neurovascular surgery in neurosurgery, with life-long continuing professional development in neuroendovascular surgery administered through a Maintenance of Certification (MOC) examination by the Neuroendovascular Surgery Fellowship Review Committee (NESAC), which was formed through collaborative efforts of the American Board of Neurosurgical Specialty (ABNS), American Board of Radiology (ABR), and the American Board of Psychiatry and Neurology (ABPN) (64,65).
Physicians seeking fellowship opportunities in neuroendovascular surgery must complete their preliminary specialty training, which includes an ACGME-approved residency in one of the three specialties, eligibility for certification, and completion MOC requirements by their respective boards. Neurologists and radiologists must also undertake ACGME-accredited fellowships in addition, with graduating residents from all three specialties eligible to enter the second year of the fellowship program if the required competencies are met (Table 2). The neuroendovascular fellowship follows for 24 months, where a minimum of 250 interventions are required as the primary operator (66). Open cerebrovascular fellowships are currently open to neurosurgeons, with a minimum of 80 interventions required to be completed during the 12-month fellowship program (Table 3). The ABNS offers a Recognition of Focused Practice (RFP) in CNS Endovascular Surgery clinical practice pathway for neurosurgeons, neurologists, and radiologists to obtain formal credentialing based on their professional experience and dedication to neuroendovascular care (67). Experienced physicians who may not have completed an accredited fellowship but have demonstrated substantial expertise in neuroendovascular procedures are eligible. To qualify, candidates must dedicate at least 50% of their clinical practice to the evaluation, management, and treatment of cerebrovascular diseases. Applicants are required to submit a detailed case log documenting at least 100 endovascular procedures and evidence of serving as the primary operator for a minimum of 250 interventional cases within the past two years, meeting the same procedure type benchmarks specified in the accredited fellowships (Table 3). Additionally, candidates must hold board certification through their respective specialty boards (ABNS, ABPN, or ABR), possess valid licensure and credentials, and achieve a passing score on the CNS Endovascular RFP written examination.
Table 2
Pathway | Prerequisites for entry | Direct 2nd-year entry eligibility |
---|---|---|
Radiology | ||
Pathway 1 | Completed ACGME- or AOA-approved diagnostic radiology residency | No |
Completed ACGME- or AOA-approved neuroradiology fellowship | ||
Pathway 2 | Completed ACGME- or AOA-approved diagnostic radiology residency | Yes |
Completed ACGME- or AOA-approved neuroradiology fellowship | ||
Six months of clinical rotations in neurological surgery, vascular neurology, or neurointensive care during PGY-5 and PGY-6 | ||
Performed at least 200 neuroangiograms under supervision | ||
Pathway 3 | Completed ACGME- or AOA-approved interventional radiology residency | Yes |
Completed ACGME- or AOA-approved neuroradiology fellowship | ||
Pathway 4 | Completed ACGME- or AOA-approved interventional radiology residency | Yes |
Completed ACGME- or AOA-approved neuroradiology fellowship | ||
Six months of clinical rotations in neurological surgery, vascular neurology, or neurointensive care during PGY-5, PGY-6, and PGY-7 | ||
Performed at least 200 neuroangiograms under supervision | ||
Neurological surgery | ||
Pathway 1 | Completed ACGME- or AOA-approved neurological surgery residency | Yes |
Preparatory year in neuroradiology with basic radiographic skills, 200 diagnostic neuroangiograms, angiographic device use, complication management, and understanding noninvasive neurovascular imaging | ||
Neurology | ||
Pathway 1 | Completed ACGME- or AOA-approved child neurology or neurology residency | Yes |
Completed ACGME- or AOA-approved fellowship in vascular neurology or neurocritical care | ||
Preparatory year in neuroradiology with basic radiographic skills, 200 diagnostic neuroangiograms, angiographic device use, complication management, and understanding noninvasive neurovascular imaging |
ACGME, Accreditation Council for Graduate Medical Education; AOA, American Osteopathic Association; PGY, postgraduate year.
Table 3
Procedure type/case | Min. cases required |
---|---|
Open neurovascular fellowships | |
Craniotomy for aneurysm | 25 |
Vascular malformations (AVM, dAVF, cavernous malformations) | 25 |
Craniotomy or MIS (endoscopy) for ICH | 10 |
STA-MCA bypass or EDAS | 10 |
CEA or TCAR | 10 |
Fellow total | 100 |
Neuroendovascular fellowships | |
Total aneurysms (ruptured and unruptured) | 40 |
Ruptured aneurysms | 10 |
Intracranial embolization, excluding MMA (AVMs, AVFs, tumors) | 20 |
Intracranial or extracranial stent placements or angioplasty (non-aneurysmal) | 20 |
Acute ischemic stroke treatments | 40 |
Head and neck embolization—excluding MMA | 15 |
Spinal angiograms and/or embolization | 5 |
MMA embolization | – |
Fellow total | 250 |
CAST, Committee on Advanced Subspecialty Training; AVM, arteriovenous malformation; AVF, arteriovenous fistula; dAVF, dural AVF; MIS, minimally invasive surgery; ICH, intracerebral hemorrhage; STA, superficial temporal artery; MCA, middle cerebral artery; EDAS, encephaloduroarteriosynangiosis; CEA, carotid endarterectomy; TCAR, transcarotid artery revascularization; MMA, middle meningeal artery; Min., minimum.
In the U.S., neurosurgery residents may also complete a year of enfolded neurovascular fellowship prior to residency graduation, with the remainder of the training undertaken following graduation. A recent survey reported a total of 57 enfolded endovascular fellowships and 13 enfolded open vascular fellowships available to residents from 91 accredited programs across the country (68). Given that CAST requires these fellowships to be taken no earlier than the sixth year of training, 24 CAST-accredited neuroendovascular and 2 open vascular programs are available to residents in their sixth year, while 19 endovascular and 7 open vascular programs are restricted for residents in their seventh year (65,68). As of 2024, the JSCVS lists 17 active programs offering open surgery fellowships in the U.S., with a maximum of 18 positions across all programs each year. On the other hand, neuroendovascular fellowships have seen a marked rise in number, with 117 positions seen across 84 programs (Figure 2, Table 4, Tables S1,S2) (69).
Table 4
Variables | Open vascular fellowships | Neuroendovascular fellowships | Total |
---|---|---|---|
Number of programs | |||
Northeast | 2 | 26 | 28 |
Midwest | 4 | 19 | 23 |
South | 5 | 26 | 31 |
West | 6 | 13 | 19 |
Total | 17 | 84 | 101 |
Number of positions | |||
Northeast | 3 | 38 | 41 |
Midwest | 3 | 23 | 26 |
South | 4 | 35 | 39 |
West | 8 | 21 | 29 |
Total | 18 | 117 | 135 |
JSCVS, Joint Section of the Cerebrovascular Surgery; AANS, American Association of Neurological Surgery; CNS, Congress of Neurological Surgeons.
Training methods in neurovascular surgery fellowships
Neurovascular training programs have a structured curriculum that incorporates competency-based learning, clinical responsibilities, formal teaching conferences, simulation-based training, multidisciplinary educational activities (e.g., case presentations, morbidity and mortality conferences), and direct participation of patient care through a required minimum number of interventional procedures (66). Advances in training have focused on simulation-based education, where neurovascular fellows maintain required competencies using surgical devices in a range of clinical scenarios at no risk to patients. For neuroendovascular interventions, trainees may use simulated catheters, wires, and stents, or may use synthetic vascular models or anesthetized animals to practice procedures. Microsurgical simulations are particularly important at this time given the eroding case volume of these surgeries. By use of simulated devices like aneurysm clips, or vascular models provided through three-dimensional (3D) printing methods, synthetic models, virtual reality (VR) programs, perfused cadavers, and human placental tissue, open neurovascular fellows can improve both surgical planning and technical skills for complex open procedures (70). In addition to rehearsing procedures, simulation-based learning can be used to set performance benchmarks for fellows (71).
Neurovascular fellows undergo a variety of assessment methods throughout their training, with case logs, objective performance evaluation, regular feedback from faculty during rotations, and final program evaluation being commonplace.
Given the established correlation between case volume and competency, the shrinking volume of open neurovascular cases has led to an emphasis on simulation-based training, didactics, and skilled tasks (70). The decrease in case volume has also been met with the abolishment of the minimum number of open procedures required for fellowship graduation, with a total case volume for vascular lesions set at 50. This may negatively impact open neurovascular fellows who may very well graduate without the skills necessary to sustain independent practice (72). Despite the pre-exquisites for neuroendovascular training, it is reported that most neurosurgery residency graduates lack the fundamental skills for a neuroendovascular fellowship, with most graduates unable to perform femoral access and closure, as well as lacking critical neurovascular anatomy knowledge (6). To mitigate this problem, longitudinal programs have been recommended in neurosurgery residency to better prepare residents for neurovascular fellowship, beginning from foundational neuroanatomical training in the first training year (postgraduate year 1) to the performance of supervised diagnostic and therapeutic angiograms in the senior years (postgraduate years 5–7) (73).
The American neurovascular surgery workforce
The current American neurovascular surgery workforce is expanding, especially in neuroendovascular surgery. The number of neurosurgeons, neurologists, and radiologists providing neuroendovascular interventions keep increasing year by year, with a recent analysis demonstrating the highest growth among neurologists. As of 2019 (most recent published data), around 12,000 neuroendovascular procedures were provided by 109 neurologists, 175 neurosurgeons, and 223 radiologists (4). The number of fellowship programs are also increasing to meet the demands of the American population: the number of open fellowships is listed as 11 in 2023 by CAST, increasing to 17 in 2024 as listed by the JSCVS of the AANS/CNS. During the same time, one additional neuroendovascular program has been listed in 2024 from the 83 in the previous year (69,74,75).
The way forward for American neurovascular surgery
The future of neurovascular surgery in the U.S. is poised for exciting times, towards personalized and minimally invasive interventions. It is expected that an increase in neuroendovascular procedures and workforce will make open neurovascular surgery difficult to sustain as dual-trained vascular neurosurgeons begin to retire. However, this special subspecialty, which upholds the utmost creativity and dexterity, can be preserved through a holistic strategy that encompasses collaborative efforts with other neurovascular interventionists, a seamless patient referral pipeline, the facilities of academic medical centers, streamlined healthcare systems, and advancing technologies (54). We have also seen the emergence of technological advances, with some in their infancy, that would further propel neurovascular surgery. These include AI, cerebrovascular robots, advanced training simulators, and brain-computer interfaces (BCIs).
AI in neurovascular surgery
AI algorithms have been used to predict the risk factors of, diagnose, select best treatments for, and predict outcomes following treatment of neurovascular conditions. Machine learning (ML) and deep learning (DL) techniques have shown promise in diagnosing intracranial aneurysms, predicting their rupture risk, and in predicting outcomes following aneurysmal subarachnoid hemorrhage (76). ML has also been used to predict with a high degree of accuracy the outcomes of AVM embolization (77). This evolving field serves to provide advanced complementary tools in neurovascular interventions, aiding in our understanding of neurovascular disease natural history, improved patient selection, and prompting appropriate patient monitoring (78). In addition to predicting neurovascular disease course, AI can also learn the motions of the neurovascular interventionalist, mimicking them and setting a benchmark for proficiency training. This has been demonstrated using a DL algorithm to track the hand motions of an open neurovascular surgeon’s technique during microvascular anastomosis (79), as well as in mechanical thrombectomy techniques for stroke (71).
The emergence of cerebrovascular robotics
Robots have the potential to transform the American neurovascular practice given their advantages of offering an enhanced, precise, and scalable approach to cerebrovascular interventions. The use of robots in neuroendovascular surgery takes inspiration from their successful implementation in interventional cardiology and radiology (80). Robotic systems such as the CorPath GRX have demonstrated promising applications in neuroendovascular settings, showing their capability in navigating intricate cerebrovascular anatomy, placing coils and stents, and performing thrombectomies in preclinical and limited contexts (81). Other robotic applications have involved the use of auto-navigation operating microscopes in the treatment neurovascular malformations (82), robotic-assisted diagnostic angiography (83,84), and robotic-assisted intracranial aneurysm clipping (85). The benefits of these technologies include increased precision through tremor-free, fine-scale movements, and enhanced control that reduces operator fatigue, both of which are critical in managing complex neurovascular procedures. These systems may also bridge disparities in the distribution of neurovascular services across the U.S., especially in cases where rapid endovascular treatment of acute ischemic stroke is required, by facilitating teleoperated procedures in a timely fashion (86). Given the declining number of open neurovascular case volumes and interventionists, robotic systems may enhance surgical training through telementoring, enabling senior specialists to guide trainees in real-time and take over if complications arise. Full integration of robotic systems in neurovascular surgery may be limited by their high costs. However, these units have the potential to become indispensable tools in neurovascular surgical practice, following continuous technological advancements and furth er clinical trials to validate their safety and efficacy.
AR platforms in neurovascular surgery
VR and extended reality (XR) hold promise for the future of neurovascular surgery through enhancing education and intraoperative practice. The use of VR platforms in neurovascular training allows for immersive, risk-free stimulations where trainees can practice complex procedures, improving their technical skills and confidence. Platforms like the Angio Mentor (for diagnostic angiography, coiling, and thrombectomy) and Mentice VIST (for diagnostic angiography and thrombectomy) have demonstrated reduced procedure times, contrast use, and fluoroscopy exposure, especially for early-stage trainees (87-90). Additionally, VR and XR can be used in preoperative planning to create patient-specific, 3D models from high-resolution imaging, allowing the better anatomical visualization and improved selection of surgical approaches (91).
Intraoperative applications of AR and mixed reality (MR) in overlaying real-time imaging to provide intraoperative guidance can be used in telementoring, enhancing learning and reducing geographic disparities in neurosurgical training. Challenges in the use of VR platforms exist, such as tool tracking accuracy issues, necessitating further development to enable incorporation into clinical education and practice (92). Nonetheless, these platforms exhibit great potential to standardize neurovascular training, improve surgical outcomes, and increase access to expert guidance.
Neuroendovascular modulation
The integration of BCIs into neuroendovascular techniques is a groundbreaking evolution in neurovascular surgery, providing untapped possibilities for minimally invasive brain modulation. The Stentrode, a transvenous stent-electrode system designed for chronic monitoring of sensorimotor cortical neural activity, is delivered endovascularly via catheter through the superior sagittal sinus (93). This approach provides a less invasive alternative to traditional intracranial implants while maintaining high temporal and spatial resolution. Initial animal studies and human trials have demonstrated the feasibility and safety of the Stentrode, with promising results in terms of recording cerebral activity and facilitating neural signal decoding for practical use (94,95). With demonstrated success in enabling patients with amyotrophic lateral sclerosis perform daily tasks (96), incorporation of BCI technology into neuroendovascular practice has the potential to revolutionize functional neurosurgery, extending their applications to more complex functions in patients following spinal cord injury or stroke.
Neurovascular surgery in other high-income countries
The neurovascular surgery landscape in Europe contrasts that of the U.S., shaped by diverse legislative frameworks, training pathways, and interdisciplinary collaboration practices. While European countries have made significant progress in integrating neurosurgeons into the endovascular field, substantial variation exists in the accessibility and structure of training programs. For instance, several European nations mandate radiology training or specific fellowship programs for neurosurgeons to gain endovascular expertise, with some countries requiring additional subspecialty certification in radiology or invasive radiology (97). Despite these advancements, resistance from radiologists and institutional barriers often limit the development of “hybrid” neurosurgical practices, particularly in countries where neurosurgeons are legally restricted from performing endovascular procedures (98). Gaps in access to training, accreditation, and endovascular services remain pronounced in Europe, limiting equitable care delivery. Recent efforts, such as the European Diploma of Interventional Neuroradiology (EDIINR), mark significant progress toward standardizing training and credentialing pathways, though challenges persist in ensuring widespread adoption and alignment across national healthcare systems.
The field of neurovascular surgery has rapidly evolved in Japan, exemplified by landmark neuroendovascular trials and registries, such as the Japanese Registry of Neuroendovascular Therapy (JR-NET) (99). One report on neuroendovascular training in Japan shows a structured three-year program that emphasizes mastering preoperative assessments, strategic planning, operative techniques, and postoperative care, alongside simulation-based training using animal models (100). Trainees must pass a rigorous board examination to qualify as independent neuroendovascular interventionists. In Korea, the Society of Korean Endovascular Neurosurgeons (SKEN) Certification Committee was established in 2010, developing rigorous standards for personal, hospital, and training institution certification, which were first implemented in 2013 (101). To achieve certification as an endovascular neurosurgeon, candidates must complete at least one year of training post-board certification, perform a minimum of 150 diagnostic cerebral angiographies, and actively assist in 80 endovascular procedures, including cases of aneurysm embolization, angioplasty, and intra-arterial thrombolysis. SKEN also mandates that certified hospitals maintain neurointensive care units, open neurosurgical backup, and well-equipped facilities, with training institutions performing annual case volumes of at least 80 endovascular procedures per year.
Limitations and conclusions
This review highlights several advancements and challenges in neurovascular surgery; however, certain limitations must be acknowledged. First, the heterogeneity of the included studies limits the generalizability of findings, particularly regarding patient outcomes and socioeconomic disparities. Second, biases related to geographic differences in access to screening and care, as well as patient adherence to recommended treatments, may influence interpretations. Additionally, while we provide a forward-looking discussion on emerging technologies, their clinical feasibility, cost-effectiveness, and scalability remain areas requiring further validation. Despite these limitations, we provide a robust foundation for understanding the current state and future trajectory of neurovascular surgery in the U.S.
Neurovascular surgery in the U.S. stands at a pivotal juncture, balancing an increased reliance on endovascular techniques with the need to sustain open surgical competencies. To address these evolving demands, a holistic approach that incorporates advanced technologies is essential in both training and clinical practice. Through multispecialty collaboration and technological integration, the U.S. neurovascular community can overcome current challenges in access, workforce training, and case distribution, ensuring that both endovascular and microsurgical treatments are available to meet diverse patient needs.
Acknowledgments
Funding: None.
Footnote
Provenance and Peer Review: This article was commissioned by the Guest Editor (Christos Tolias) for the series “State of Neurovascular Surgery. The Way Forward” published in AME Surgical Journal. The article has undergone external peer review.
Peer Review File: Available at https://asj.amegroups.com/article/view/10.21037/asj-24-45/prf
Conflicts of Interest: All authors have completed the ICMJE uniform disclosure form (available at https://asj.amegroups.com/article/view/10.21037/asj-24-45/coif). The series “State of Neurovascular Surgery. The Way Forward” was commissioned by the editorial office without any funding or sponsorship. The authors have no other conflicts of interest to declare.
Ethical Statement: The authors are accountable for all aspects of the work in ensuring that questions related to the accuracy or integrity of any part of the work are appropriately investigated and resolved.
Open Access Statement: This is an Open Access article distributed in accordance with the Creative Commons Attribution-NonCommercial-NoDerivs 4.0 International License (CC BY-NC-ND 4.0), which permits the non-commercial replication and distribution of the article with the strict proviso that no changes or edits are made and the original work is properly cited (including links to both the formal publication through the relevant DOI and the license). See: https://creativecommons.org/licenses/by-nc-nd/4.0/.
References
- Britz G. An overview of neurovascular disease management. Methodist Debakey Cardiovasc J 2014;10:212-3. [Crossref] [PubMed]
- Tong X, Yang Q, Ritchey MD, et al. The Burden of Cerebrovascular Disease in the United States. Prev Chronic Dis 2019;16:E52. [Crossref] [PubMed]
- Riina HA. Neuroendovascular surgery. J Neurosurg 2019;131:1690-701. [Crossref] [PubMed]
- Steiger K, Singh R, Fox WC, et al. Procedural, workforce, and reimbursement trends in neuroendovascular procedures. J Neurointerv Surg 2023;15:909-13. [Crossref] [PubMed]
- Kwok CS, Gillani SA, Bains NK, et al. Mechanical thrombectomy in patients with acute ischemic stroke in the USA before and after time window expansion. J Neurointerv Surg 2024;16:447-52. [Crossref] [PubMed]
- Chalouhi N, Zanaty M, Tjoumakaris S, et al. Preparedness of neurosurgery graduates for neuroendovascular fellowship: a national survey of fellowship programs. J Neurosurg 2015;123:1113-9. [Crossref] [PubMed]
- de Donato G, Setacci F, Chisci E, et al. Open vascular surgery as a starting point for endovascular surgery. J Cardiovasc Surg (Torino) 2011;52:9-16. [PubMed]
- Guglielmi G. The beginning and the evolution of the endovascular treatment of intracranial aneurysms: from the first catheterization of brain arteries to the new stents. J Neurointerv Surg 2009;1:53-5. [Crossref] [PubMed]
- Gory B, Spiotta AM, Mangiafico S, et al. PulseRider Stent-Assisted Coiling of Wide-Neck Bifurcation Aneurysms: Periprocedural Results in an International Series. AJNR Am J Neuroradiol 2016;37:130-5. [Crossref] [PubMed]
- De Leacy RA, Fargen KM, Mascitelli JR, et al. Wide-neck bifurcation aneurysms of the middle cerebral artery and basilar apex treated by endovascular techniques: a multicentre, core lab adjudicated study evaluating safety and durability of occlusion (BRANCH). J Neurointerv Surg 2019;11:31-6. [Crossref] [PubMed]
- Arthur AS, Molyneux A, Coon AL, et al. The safety and effectiveness of the Woven EndoBridge (WEB) system for the treatment of wide-necked bifurcation aneurysms: final 12-month results of the pivotal WEB Intrasaccular Therapy (WEB-IT) Study. J Neurointerv Surg 2019;11:924-30. [Crossref] [PubMed]
- Shao MM, White TG, Bassett JB, et al. Intrasaccular Treatment of Intracranial Aneurysms: A Comprehensive Review. J Clin Med 2024;13:6162. [Crossref] [PubMed]
- Becske T, Kallmes DF, Saatci I, et al. Pipeline for uncoilable or failed aneurysms: results from a multicenter clinical trial. Radiology 2013;267:858-68. [Crossref] [PubMed]
- Mawad ME, Cekirge S, Ciceri E, et al. Endovascular treatment of giant and large intracranial aneurysms by using a combination of stent placement and liquid polymer injection. J Neurosurg 2002;96:474-82. [Crossref] [PubMed]
- Briganti F, Leone G, Marseglia M, et al. Endovascular treatment of cerebral aneurysms using flow-diverter devices: A systematic review. Neuroradiol J 2015;28:365-75. [Crossref] [PubMed]
- Cappuzzo JM, Monteiro A, Taylor MN, et al. First U.S. Experience Using the Pipeline Flex Embolization Device with Shield Technology for Treatment of Intracranial Aneurysms. World Neurosurg 2022;159:e184-91. [Crossref] [PubMed]
- Lawton MT. Basilar apex aneurysms: surgical results and perspectives from an initial experience. Neurosurgery 2002;50:1-8; discussion 8-10. [PubMed]
- Siddiqui AH, Abla AA, Kan P, et al. Panacea or problem: flow diverters in the treatment of symptomatic large or giant fusiform vertebrobasilar aneurysms. J Neurosurg 2012;116:1258-66. [Crossref] [PubMed]
- Duffis EJ, Tank V, Gandhi CD, et al. Recent advances in neuroendovascular therapy. Clin Neurol Neurosurg 2013;115:853-8. [Crossref] [PubMed]
- Powers WJ, Rabinstein AA, Ackerson T, et al. 2018 Guidelines for the Early Management of Patients With Acute Ischemic Stroke: A Guideline for Healthcare Professionals From the American Heart Association/American Stroke Association. Stroke 2018;49:e46-e110. [Crossref] [PubMed]
- Zaidat OO, Bozorgchami H, Ribó M, et al. Primary Results of the Multicenter ARISE II Study (Analysis of Revascularization in Ischemic Stroke With EmboTrap). Stroke 2018;49:1107-15. [Crossref] [PubMed]
- Turk AS 3rd, Siddiqui A, Fifi JT, et al. Aspiration thrombectomy versus stent retriever thrombectomy as first-line approach for large vessel occlusion (COMPASS): a multicentre, randomised, open label, blinded outcome, non-inferiority trial. Lancet 2019;393:998-1008. [Crossref] [PubMed]
- Bageac DV, Gershon BS, Vargas J, et al. Comparative study of intracranial access in thrombectomy using next generation 0.088 inch guide catheter technology. J Neurointerv Surg 2022;14:390-6. [Crossref] [PubMed]
- Boggs KM, Vogel BT, Zachrison KS, et al. An inventory of stroke centers in the United States. J Am Coll Emerg Physicians Open 2022;3:e12673. [Crossref] [PubMed]
- The Joint Commission. The Joint Commission Stroke Certification Programs – Program Concept Comparison. 2021. Available online: https://www.jointcommission.org/-/media/tjc/documents/accred-and-cert/certification/certification-by-setting/stroke/dsc-stroke-grid-comparison-chart-42021.pdf
- Rennert RC, Wali AR, Steinberg JA, et al. Epidemiology, Natural History, and Clinical Presentation of Large Vessel Ischemic Stroke. Neurosurgery 2019;85:S4-8. [Crossref] [PubMed]
- Golnari P, Nazari P, Ansari SA, et al. Endovascular Thrombectomy after Large-Vessel Ischemic Stroke: Utilization, Outcomes, and Readmissions across the United States. Radiology 2021;299:179-89. [Crossref] [PubMed]
- Rai AT, Link PS, Domico JR. Updated estimates of large and medium vessel strokes, mechanical thrombectomy trends, and future projections indicate a relative flattening of the growth curve but highlight opportunities for expanding endovascular stroke care. J Neurointerv Surg 2023;15:e349-55. [Crossref] [PubMed]
- Heilman CB, Basil GW, Beneduce BM, et al. Anatomical characterization of the inferior petrosal sinus and adjacent cerebellopontine angle cistern for development of an endovascular transdural cerebrospinal fluid shunt. J Neurointerv Surg 2019;11:598-602. [Crossref] [PubMed]
- Malek AM, Beneduce BM, Heilman CB. Endovascular Shunting for Communicating Hydrocephalus Using a Biologically Inspired Transdural Cerebrospinal Fluid Valved eShunt® Implant. Neurosurg Clin N Am 2024;35:379-87. [Crossref] [PubMed]
- Malek AM, Heilman CB. Response to letter to editor regarding 'Anatomical characterization of the inferior petrosal sinus and adjacent cerebellopontine angle cistern for development of an endovascular transdural cerebrospinal fluid shunt'. J Neurointerv Surg 2021;13:e25. [Crossref] [PubMed]
- Nouri A, Gondar R, Schaller K, et al. Chronic Subdural Hematoma (cSDH): A review of the current state of the art. Brain Spine 2021;1:100300. [Crossref] [PubMed]
- Link TW, Rapoport BI, Paine SM, et al. Middle meningeal artery embolization for chronic subdural hematoma: Endovascular technique and radiographic findings. Interv Neuroradiol 2018;24:455-62. [Crossref] [PubMed]
- Sahyouni R, Goshtasbi K, Mahmoodi A, et al. Chronic Subdural Hematoma: A Historical and Clinical Perspective. World Neurosurg 2017;108:948-53. [Crossref] [PubMed]
- Srivatsan A, Mohanty A, Nascimento FA, et al. Middle Meningeal Artery Embolization for Chronic Subdural Hematoma: Meta-Analysis and Systematic Review. World Neurosurg 2019;122:613-9. [Crossref] [PubMed]
- Pierot L, Cognard C, Herbreteau D, et al. Endovascular treatment of brain arteriovenous malformations using a liquid embolic agent: results of a prospective, multicentre study (BRAVO). Eur Radiol 2013;23:2838-45. [Crossref] [PubMed]
- Piechowiak E, Zibold F, Dobrocky T, et al. Endovascular Treatment of Dural Arteriovenous Fistulas of the Transverse and Sigmoid Sinuses Using Transarterial Balloon-Assisted Embolization Combined with Transvenous Balloon Protection of the Venous Sinus. AJNR Am J Neuroradiol 2017;38:1984-9. [Crossref] [PubMed]
- Loh Y, Duckwiler GROnyx Trial Investigators. A prospective, multicenter, randomized trial of the Onyx liquid embolic system and N-butyl cyanoacrylate embolization of cerebral arteriovenous malformations. Clinical article. J Neurosurg 2010;113:733-41. [Crossref] [PubMed]
- Samaniego EA, Dabus G, Meyers PM, et al. Most Promising Approaches to Improve Brain AVM Management: ARISE I Consensus Recommendations. Stroke 2024;55:1449-63. [Crossref] [PubMed]
- Chen CJ, Norat P, Ding D, et al. Transvenous embolization of brain arteriovenous malformations: a review of techniques, indications, and outcomes. Neurosurg Focus 2018;45:E13. [Crossref] [PubMed]
- Korkmazer B, Kocak B, Tureci E, et al. Endovascular treatment of carotid cavernous sinus fistula: A systematic review. World J Radiol 2013;5:143-55. [Crossref] [PubMed]
- Tsai YH, Wong HF, Chen YL, et al. Transarterial Embolization of Direct Carotid Cavernous Fistulas with the Double-balloon Technique. Interv Neuroradiol 2008;14:13-7. [Crossref] [PubMed]
- Alaraj A, Wallace A, Dashti R, et al. Balloons in endovascular neurosurgery: history and current applications. Neurosurgery 2014;74:S163-90. [Crossref] [PubMed]
- Texakalidis P, Tzoumas A, Xenos D, et al. Carotid cavernous fistula (CCF) treatment approaches: A systematic literature review and meta-analysis of transarterial and transvenous embolization for direct and indirect CCFs. Clin Neurol Neurosurg 2021;204:106601. [Crossref] [PubMed]
- Pradeep N, Nottingham R, Kam A, et al. Treatment of post-traumatic carotid-cavernous fistulas using pipeline embolization device assistance. BMJ Case Rep 2015;2015:bcr2015011786. [Crossref] [PubMed]
- Baharvahdat H, Ooi YC, Kim WJ, et al. Updates in the management of cranial dural arteriovenous fistula. Stroke Vasc Neurol 2020;5:50-8. [Crossref] [PubMed]
- Natarajan SK, Ghodke B, Kim LJ, et al. Multimodality treatment of intracranial dural arteriovenous fistulas in the Onyx era: a single center experience. World Neurosurg 2010;73:365-79. [Crossref] [PubMed]
- Dinkin MJ, Patsalides A. Idiopathic Intracranial Venous Hypertension: Toward a Better Understanding of Venous Stenosis and the Role of Stenting in Idiopathic Intracranial Hypertension. J Neuroophthalmol 2023;43:451-63. [Crossref] [PubMed]
- Starke RM, Wang T, Ding D, et al. Endovascular Treatment of Venous Sinus Stenosis in Idiopathic Intracranial Hypertension: Complications, Neurological Outcomes, and Radiographic Results. ScientificWorldJournal 2015;2015:140408. [Crossref] [PubMed]
- Spitze A, Malik A, Lee AG. Surgical and endovascular interventions in idiopathic intracranial hypertension. Curr Opin Neurol 2014;27:69-74. [Crossref] [PubMed]
- Fiani B, Kondilis A, Doan T, et al. Venous sinus stenting for intractable pulsatile tinnitus: A review of indications and outcomes. Surg Neurol Int 2021;12:81. [Crossref] [PubMed]
- Lawton MT. A Lasting Future for Open Vascular Neurosurgery. Neurosurg Clin N Am 2022;33:xiii-xiv. [Crossref] [PubMed]
- Mazur MD, Taussky P, Park MS, et al. Contemporary endovascular and open aneurysm treatment in the era of flow diversion. J Neurol Neurosurg Psychiatry 2018;89:277-86. [Crossref] [PubMed]
- Lawton MT, Lang MJ. The future of open vascular neurosurgery: perspectives on cavernous malformations, AVMs, and bypasses for complex aneurysms. J Neurosurg 2019;130:1409-25. [Crossref] [PubMed]
- Quiñones-Hinojosa A, Du R, Lawton MT. Revascularization with saphenous vein bypasses for complex intracranial aneurysms. Skull Base 2005;15:119-32. [Crossref] [PubMed]
- Tayebi Meybodi A, Huang W, Benet A, et al. Bypass surgery for complex middle cerebral artery aneurysms: an algorithmic approach to revascularization. J Neurosurg 2017;127:463-79. [Crossref] [PubMed]
- Meling TR, Sorteberg A, Bakke SJ, et al. Blood blister-like aneurysms of the internal carotid artery trunk causing subarachnoid hemorrhage: treatment and outcome. J Neurosurg 2008;108:662-71. [Crossref] [PubMed]
- Bowers CA, Taussky P, Park MS, et al. Rescue microsurgery with bypass and stent removal following Pipeline treatment of a giant internal carotid artery terminus aneurysm. Acta Neurochir (Wien) 2015;157:2071-5. [Crossref] [PubMed]
- Potts MB, Lau D, Abla AA, et al. Current surgical results with low-grade brain arteriovenous malformations. J Neurosurg 2015;122:912-20. [Crossref] [PubMed]
- Lawton MTUCSF Brain Arteriovenous Malformation Study Project. Spetzler-Martin Grade III arteriovenous malformations: surgical results and a modification of the grading scale. Neurosurgery 2003;52:740-8; discussion 748-9. [Crossref] [PubMed]
- Spetzler RF, Ponce FA. A 3-tier classification of cerebral arteriovenous malformations. Clinical article. J Neurosurg 2011;114:842-9. [Crossref] [PubMed]
- Darsaut TE, Magro E, Bojanowski MW, et al. Surgical treatment of brain arteriovenous malformations: clinical outcomes of patients included in the registry of a pragmatic randomized trial. J Neurosurg 2023;138:891-9. [Crossref] [PubMed]
- Sur S, Menaker SA, Alvarez C, et al. Multimodal Management of Carotid-Cavernous Fistulas. World Neurosurg 2020;133:e796-803. [Crossref] [PubMed]
- Linfante I. The long winding road towards certification in neuroendovascular surgery. J Neurointerv Surg 2016;8:441-2. [Crossref] [PubMed]
- Day AL, Siddiqui AH, Meyers PM, et al. Training Standards in Neuroendovascular Surgery: Program Accreditation and Practitioner Certification. Stroke 2017;48:2318-25. [Crossref] [PubMed]
- ACGME. ACGME Program Requirements for Graduate Medical Education in Neuroendovascular Intervention. 2022. Available online: https://www.acgme.org/globalassets/pfassets/programrequirements/163_neuroendovascularintervention_2023.pdf
- The American Board of Neurological Surgery. RFP: CNS Neuroendovascular Surgery. 2024. Available online: https://abns.org/rfp-cnsendo/
- Kolcun JPG, Mazza JM, Pawlowski KD, et al. The Evolving Role of Postgraduate Year 7 in Neurological Surgery Residency. Neurosurgery 2024;94:350-7. [PubMed]
- AANS/CNS Cerebrovascular Section. Fellowships. 2024. Available online: https://cvsection.org/education/fellowships/fellowships/
- Scullen T, Milburn J, Mathkour M, et al. Training Cerebrovascular and Neuroendovascular Surgery Residents: A Systematic Literature Review and Recommendations. Ochsner J 2024;24:36-46. [Crossref] [PubMed]
- Liebig T, Holtmannspötter M, Crossley R, et al. Metric-Based Virtual Reality Simulation: A Paradigm Shift in Training for Mechanical Thrombectomy in Acute Stroke. Stroke 2018;49:e239-42. [Crossref] [PubMed]
- Fredrickson VL, Strickland BA, Ravina K, et al. State of the Union in Open Neurovascular Training. World Neurosurg 2019;122:e553-60. [Crossref] [PubMed]
- Kumarapuram S, Sreenivasan S, Roychowdhury S, et al. The advent of endovascular neurosurgery, its impact on neurosurgery residency training, and the making of a vascular neurosurgeon. J Neurosurg 2023;139:292-7. [Crossref] [PubMed]
- CAST: The Committee on Advanced Subspecialty Training. CAST accredited fellowship programs in Cerebrovascular. 2023. Available online: https://sns-cast.org/cast-approved-fellowship-programs-in-cerebrovascular-neurological-surgery/
- CAST: The Committee on Advanced Subspecialty Training. CAST accredited fellowship programs in Neuroendovascular. 2023. Available online: https://sns-cast.org/cast-approved-fellowship-programs-in-cns-endovascular-surgery/
- Mensah E, Pringle C, Roberts G, et al. Deep Learning in the Management of Intracranial Aneurysms and Cerebrovascular Diseases: A Review of the Current Literature. World Neurosurg 2022;161:39-45. [Crossref] [PubMed]
- Asadi H, Kok HK, Looby S, et al. Outcomes and Complications After Endovascular Treatment of Brain Arteriovenous Malformations: A Prognostication Attempt Using Artificial Intelligence. World Neurosurg 2016;96:562-569.e1. [Crossref] [PubMed]
- Senders JT, Staples PC, Karhade AV, et al. Machine Learning and Neurosurgical Outcome Prediction: A Systematic Review. World Neurosurg 2018;109:476-486.e1. [Crossref] [PubMed]
- Gonzalez-Romo NI, Hanalioglu S, Mignucci-Jiménez G, et al. Quantification of motion during microvascular anastomosis simulation using machine learning hand detection. Neurosurg Focus 2023;54:E2. [Crossref] [PubMed]
- Menaker SA, Shah SS, Snelling BM, et al. Current applications and future perspectives of robotics in cerebrovascular and endovascular neurosurgery. J Neurointerv Surg 2018;10:78-82. [Crossref] [PubMed]
- Britz GW, Tomas J, Lumsden A. Feasibility of Robotic-Assisted Neurovascular Interventions: Initial Experience in Flow Model and Porcine Model. Neurosurgery 2020;86:309-14. [Crossref] [PubMed]
- Bohl MA, Oppenlander ME, Spetzler R. A Prospective Cohort Evaluation of a Robotic, Auto-Navigating Operating Microscope. Cureus 2016;8:e662. [Crossref] [PubMed]
- Murayama Y, Irie K, Saguchi T, et al. Robotic digital subtraction angiography systems within the hybrid operating room. Neurosurgery 2011;68:1427-32; discussion 1433. [Crossref] [PubMed]
- Lu WS, Xu WY, Pan F, et al. Clinical application of a vascular interventional robot in cerebral angiography. Int J Med Robot 2016;12:132-6. [Crossref] [PubMed]
- Kato T, Okumura I, Song SE, et al. Multi-section continuum robot for endoscopic surgical clipping of intracranial aneurysms. Med Image Comput Comput Assist Interv 2013;16:364-71.
- Grotta JC, Lyden P, Brott T. Rethinking Training and Distribution of Vascular Neurology Interventionists in the Era of Thrombectomy. Stroke 2017;48:2313-7. [Crossref] [PubMed]
- Dardick J, Allen S, Scoco A, et al. Virtual reality simulation of neuroendovascular intervention improves procedure speed in a cohort of trainees. Surg Neurol Int 2019;10:184. [Crossref] [PubMed]
- Kreiser K, Ströber L, Gehling KG, et al. Simulation Training in Neuroangiography-Validation and Effectiveness. Clin Neuroradiol 2021;31:465-73. [Crossref] [PubMed]
- Elsawaf Y, Rennert RC, Steinberg JA, et al. Simulator Training for Endovascular Neurosurgery. J Vis Exp 2020; [Crossref] [PubMed]
- Schneider MS, Sandve KO, Kurz KD, et al. Metric based virtual simulation training for endovascular thrombectomy improves interventional neuroradiologists' simulator performance. Interv Neuroradiol 2023;29:577-82. [Crossref] [PubMed]
- Hendricks BK, Hartman J, Cohen-Gadol AA. Cerebrovascular Operative Anatomy: An Immersive 3D and Virtual Reality Description. Oper Neurosurg (Hagerstown) 2018;15:613-23. [Crossref] [PubMed]
- Patel SA, Covell MM, Patel S, et al. Advancing endovascular neurosurgery training with extended reality: opportunities and obstacles for the next decade. Front Surg 2024;11:1440228. [Crossref] [PubMed]
- Oxley TJ, Opie NL, John SE, et al. Minimally invasive endovascular stent-electrode array for high-fidelity, chronic recordings of cortical neural activity. Nat Biotechnol 2016;34:320-7. [Crossref] [PubMed]
- Opie NL, John SE, Rind GS, et al. Focal stimulation of the sheep motor cortex with a chronically implanted minimally invasive electrode array mounted on an endovascular stent. Nat Biomed Eng 2018;2:907-14. [Crossref] [PubMed]
- Oxley TJ, Yoo PE, Rind GS, et al. Motor neuroprosthesis implanted with neurointerventional surgery improves capacity for activities of daily living tasks in severe paralysis: first in-human experience. J Neurointerv Surg 2021;13:102-8. [Crossref] [PubMed]
- Mitchell P, Lee SCM, Yoo PE, et al. Assessment of Safety of a Fully Implanted Endovascular Brain-Computer Interface for Severe Paralysis in 4 Patients: The Stentrode With Thought-Controlled Digital Switch (SWITCH) Study. JAMA Neurol 2023;80:270-8. [Crossref] [PubMed]
- Peschillo S, Meling TR. Endovascular neurosurgery in Europe: an International Survey and future perspectives. J Neurosurg Sci 2024;68:254-9. [Crossref] [PubMed]
- Sun R, Taussky P, Levy EI, et al. Neurosurgical Endovascular Credentialing in Europe and the United Kingdom for the “Complete” Neurovascular Surgeon: The Time has Come. Neurosurgery 2024; [Crossref]
- Sakai N, Fujiwara S, Uchida K, et al. Trends and Progress in Neuroendovascular Treatment in Japan: Japanese Registry of Neuroendovascular Therapy (JR-NET) 4. Main Report. Neurol Med Chir (Tokyo) 2024;64:309-15. [Crossref] [PubMed]
- Miyachi S, Okamoto T, Kobayashi N, et al. Nagoya university training system for neuroendovascular therapists. Interv Neuroradiol 2004;10:103-6. [Crossref] [PubMed]
- Shin DS, Park SQ, Kang HS, et al. Standards for endovascular neurosurgical training and certification of the society of korean endovascular neurosurgeons 2013. J Korean Neurosurg Soc 2014;55:117-24. [Crossref] [PubMed]
Cite this article as: Mensah EO, Jokhio A, Ogilvy CS. Neurovascular surgery: a review of the way forward from the American perspective. AME Surg J 2024;4:21.